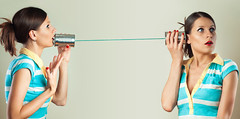
Thursday, GenBioOrg brought in Prof. Ron Weiss to speak about his work in designing biological circuits, and this crazy, sometimes hyped field of synthetic biology. Prior to Prof. Weiss's talk, while I could appreciate the idea of synthetic biology, I mostly regarded it as a somewhat foolish pursuit, on account of the amount of fundamental biology we still just do not know. In many ways, my field of computational systems biology relies on building and testing models from "Swiss cheese knowledge", where gaps prevail (e.g., protein-protein interaction networks built from yeast-two-hybrid studies with high false-positive rates, or microarray analysis suffering from from the high-dimensionality, low-sample conundrum). Thus, whatever decries the prematurity of systems biology goes doubly so for synthetic biology, for which systems biology provides a central strut. The rationale is, if you don't understand it, how can you manipulate it? Of course, as I've learned repeatedly (but have failed to generalize), "You don't need to understand the internal combustion engine to drive a car."
Well, Thursday afternoon, Prof. Weiss deftly reminded me of this reality through his combination of humility-tempered optimism, his impressive collection of proofs-of-concept, and his insight for possible applications. He presented a number of intriguing biological circuits in his talk, but I felt most excited by his work on pattern formation through synthetic inter-cellular signaling networks (behind a Nature paywall, sorry). In this work, Weiss and his colleagues created a population of "receiver" bacteria cells, which had a genetic circuit that would cause cells to fluoresce (light up green) at a moderate concentration of a molecule called acyl-homoserine lactone (AHL).
To make the receivers fluoresce within a specific concentration of AHL, Weiss and colleagues actually made the receiver cells fluoresce (i.e. "be on") by default. They then created two AHL-detection circuits with very different input thresholds: a high-detection circuit which activates in the presence of large amounts of AHL, and a low-detection circuit which activates in low amounts or in the absence of AHL. Weiss and colleagues wired both detectors to the same output: when activated, they repressed ("turned off") fluorescence. If you're familiar with electronics, you'll see that Weiss and colleagues constructed a NOR gate, where the inputs are "high AHL" and "low/no AHL". If you're a programmer, you might think of the condition for fluorescence as
if not (ahl_level > high_threshold) and not (ahl_level < low_threshold): cells.fluoresce()
Weiss and colleagues then developed "sender" cells containing a circuit that caused synthesis and secretion AHL when exposed to tetracycline. When a colony of sender cells was placed in the middle of a "lawn" of receiver cells and exposed to tetracycline, the sender cells emitted AHL, which then diffused as a radial gradient from the colony, resulting in a concentric ring of fluorescence around the sender colony, but not immediately touching it, like a bullseye. That is right by the sender colony, the AHL was highest, and so the high-detection AHL circuit shut off fluorescence and left those cells dark. A little further out, the levels of AHL that diffused from the senders was at a more moderate amount, so the high-detection and the low-detection circuits remained off, allowing those cells to fluoresce. Beyond those cells, the levels of AHL were too low, and though the high-detection circuit remained off, the low-detection circuit turned on and repressed the fluorescence, again.

While this makes for pretty pictures, taxpayers rest assured: glowing cells are only the proof of concept. This research has major implications for practical applications, for example, in stem cell research, tissue engineering, and bioengineering.
As a high school student, I felt incredibly excited to learn the answer to the question, "How can a ball of indistinguishable cells turn into a brain, limbs, skin, etc.?" The answer, as those of you with some developmental biology background know, is "Through protein gradients," and more specifically through transcription factors and their co-activators and co-repressors. Beginning with your mother's egg cell, there already existed protein gradients which pre-determined the regions that formed your head, or your feet, or your inner organs, and as your zygotic cells divided, these protein gradients begot even more protein gradients, in a beautiful choreography perfected through billions of years of evolution. This research by Prof. Weiss and his colleagues demonstrates that synthetic biology may provide a means to not only guiding stem cells (either derived from an embryo or returned to their embryo-like stage) through the difficult process of differentiating into other cell types when cued by specific protein concentrations, but also the means to create colonies of cells capable of producing protein gradients. Through a successful combination of these sender-recipient circuits, we could achieve multiple types of differentiated cells, and maybe even self-organizing tissues, all from the same culture of stem cells.
Likewise, this research has important implications in mixed cell cultures. For example, the liver is primarily composed of cells called hepatocytes, which perform most of the functions of the liver, such as detoxification, lipid homeostasis, and blood plasma production. However, by culturing hepatocytes together with another cell type found in the liver, called liver sinusoidal endothelial cells (LSECs), the hepatocytes maintain their "liver-ness" far better than when cultured alone. Weiss's research implies that we may some day be able to develop synthetic "surrogate" cells to support cells that are characteristically difficult to maintain ex vivo by providing important intercellular signals.
In terms of bioengineering applications, such as biodiesel or pharmaceutical production, a major stumbling block has been the difficulty in engineering biological systems with the biochemical capacities necessary to carry out each step necessary to manufacture a complex molecule. Weiss's research suggests growing practicality in molecule manufacturing by designing chains biological pathways that exist in separate organisms, much as the case for deep-sea vents ecosystems.
Two other profound discoveries that Weiss presented were completely counterintuitive to me: adding complexity to a biological circuit tends to 1) bring about more digital (on/off) behavior rather than analog (continuous gradient from low to high) behavior, and that coupling components tends to reduce noisiness in the circuit rather than increase it. Although I do not have time to recapitulate Prof. Weiss's demonstrations of these emergent behaviors, I encourage you to browse through his publications yourself.
The last two points I'd like to note from Prof. Weiss's talk are the following quips, which I found particularly encouraging (paraphrasing). First:
Computational simulation is absolutely central to synthetic biology. We are beyond the point where we can design biological circuits through intuition alone. —Prof. Ron WeissThis statement makes me feel validated for pursuing a background in computational biology. Second:
We've been working on a project for eight years now that we still haven't published results from. We're very close, though. It will be just another year or so. At least, that's what I tell my graduate student. And the graduate student that takes the project after she graduates. And the one after she graduates. —Prof. Ron WeissResearchers with careers as illustrious as Prof. Weiss's can come in and dazzle us grad students with tales of field-changing success, and I think this gives unreasonable and unwarranted expectations of how our own research paths should go. Certainly in my case I've felt that because I've struggled, I must not be successful, because it rarely seems the successful people struggled. It's refreshing to see an admirable figure in his field open up and show vulnerability by admitting that, even to this day, he has his struggles.
To summarize, here are my takeaway thoughts from Prof. Weiss's talk:
- The time for synthetic biology research is now.
- Researchers can engineer cell-cell communication, beginning the era of human-designed mixed cell cultures.
- Even excellent researchers struggle.